The Strano group is using advances in nanotechnology, including the engineering of new catalytic materials and active sites, to solve problems in green and sustainable chemistry. By engineering catalysts at the nanometer scale, the team has been able to generate useful products and self healing materials from greenhouse gases such as methane and carbon dioxide.
Research Overview
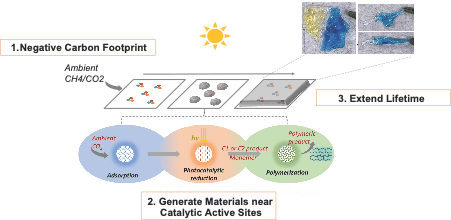
Carbon Dioxide Fixing Materials
As an initial proof of concept for CFMs, we utilized extracted plant chloroplasts as unique photocatalysts that react with atmospheric carbon dioxide using ambient solar energy to produce saccharides such as glucose, maltose, and triose phosphate. The material prototype includes enzymes to selectively convert these products into glucose. Within the material, glucose oxidase converts glucose into reactive gluconolactone, which subsequently reacts with a primary amine-functionalized acrylamide monomer, 3-aminopropyl methacrylamide (APMA), to form a continuously extending polymer matrix. This synthetic pathway is outlined in Fig. 1. After 18 hours of light exposure and atmospheric carbon dioxide at room temperature, a hydrogel-like material was observed forming around the chloroplast membrane (Fig. 1b). Additionally, physically separated hydrogels seamlessly recombined upon light exposure, demonstrating a unique self-repair property of the carbon-fixing material (Fig. 1c-e). The mechanical properties evolved over time, with the material growing measurably over 24 hours to produce a polymer matrix and repair cracks. This initial study established that CFMs are feasible and possess highly desirable material properties.
References
Methanotrophic Materials

Synthetic Methanotrophic systems that produce valuable polymer materials at ambient conditions: Anthropogenic methane emissions to the atmosphere significantly contribute to climate warming. Its concentration has tripled to 1900 ppb since pre-industrial times, making it the second largest contributor to climate warming. However, the low temperature and concentration of these emission streams make them diffic
ult to address by currently proposed methane oxidation routes, which rely on high temperatures or pressures to drive methane oxidation rapidly and efficiently. We have introduced a novel tandem catalytic system, combining alcohol oxidase with iron-modified ZSM-5 that functions as a synthetic methanotrophic system capable of partially oxidizing methane at ambient temperatures and pressures, producing chemically useful intermediates for polymer material synthesis (Fig.2A). Our results show that the methane-to-formaldehyde selectivity can exceed 90% at room temperature and 0.5 atm of CH4 and air (Fig. 2B-C). The generated formaldehyde intermediate was rapidly incorporated into a growing urea polymer, with a material growth rate exceeding 5.0 mg gcat hr-1 under ambient conditions, surpassing rates observed in many cultured methanotrophic bacteria systems (Fig.2D).
References
Lundberg, D. J.,* Kim, J.,* Tu, Y-M., Ritt, C. L. & Strano, M. S. Concerted Methane Fixation at Ambient Temperature and Pressure Mediated by an Alcohol Oxidase and Fe-ZSM-5 Catalytic Couple. Nat. Cat. (2024).