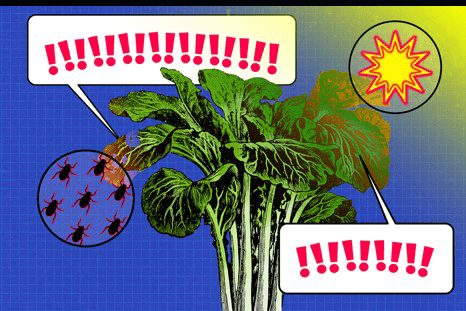
Plant Nanotechnology
Applying chemical engineering and nanotechnology to unlock the mysteries of plant systems! From understanding nanoparticle movement in living tissues to introducing the concept of plant signaling waveforms, we’re revealing how plants communicate and thrive.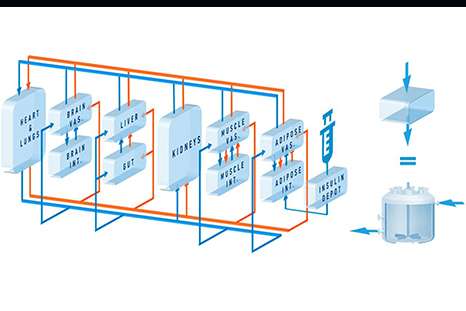
Medical Nanotechnology
Innovating solutions for oncology, diabetes, and medical imaging with new synthetic techniques like Corona Phase Molecular Recognition and advanced chemical engineering analysis of the human body.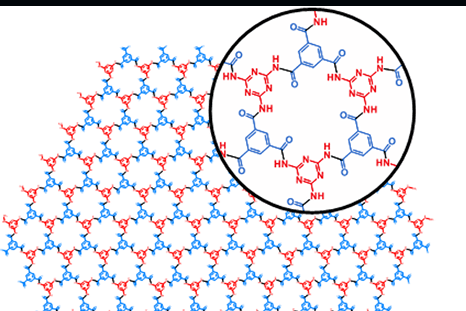
2D Polyaramids
Leveraging cutting-edge synthetic techniques and mechanistic modeling to develop materials with unique and unprecedented properties, including two-dimensionally structured polyaramids and innovative characterization tools.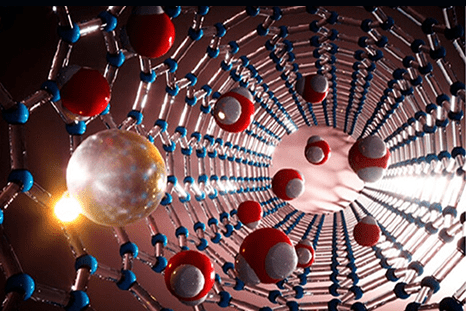
Thermodynamics and Transport of Nano-confined Fluids
Research on the behavior of 1D and 2D solid-state nanopores and nano-confined systems. Recent work has focused on nano-confined fluids as a means of understanding nano-scale thermodynamics and transport.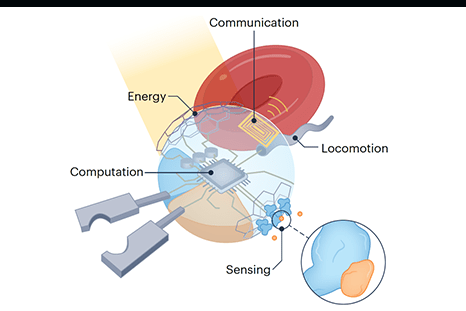
Colloidal Electronics and Robotics
Pioneering particle-sized electronic systems that push the boundaries of nanoelectronics into new realms! With a vision for colloidal robotics, we aim to create cell-sized, micron-scale machines that can operate in previously inaccessible locations.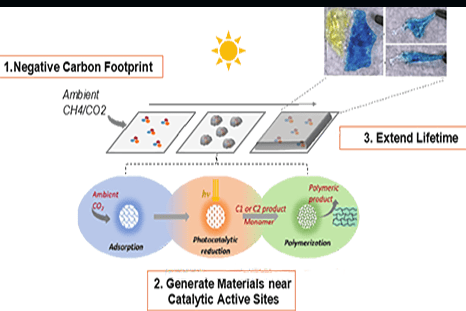